By Gregory Knoop
Money is not the only – or even the most important – measure of worth. There are other values that we hold dear such as the quality of or the impact of our work for the greater good. If we look at those criteria, we may find other value commodities to measure.
In value engineering studies, the value proposition is expressed with the following formula:
Value = function/resources
Frequently, the resource is equated to the dollar. However, there is another group of resources that should be taken into consideration and can be tracked such as measuring carbon dioxide (CO2), water (H2O), kWh, BTUs and a variety of other elements. Too often these resources are overlooked although they play an important role in international policy and diplomacy concerns.
With the U.S. on the verge of reentering the cooperative agreements of the Paris Climate Accords of 2014, we rejoin a world that is more attentive to the scarcity of water and the build-up of CO2 in the atmosphere, and that has greater sensitivity to the broader issues of anthropogenic impacts on our common home – the planet Earth. Water and carbon specifically are creating climate-related stresses in the form of warming and critical resources for human life. These challenges make it critical that a value engineering study for U.S. government agencies, especially the Department of State and Defense buildings overseas, shows other measures of value beyond dollars.
In a value study for a U.S. diplomatic project in an arid African location, the team introduced the concepts of other measures. We were able to look at many of the value alternatives regarding their impacts as represented in embodied energy and expressed in CO2 and embodied water. The team was not able to do a gross model of the project, but it is very probable that the construction of this project could generate well over 100,000 metric tons (MT, each MT= 1000Kg) of CO2 emissions and consume over 6 million liters of water. This means that changes in the design proposed as value alternatives may have compelling resource reductions in addition to those that are measured by dollars and should be considered.
One example from the recommendations was the introduction of chill-beams, which created a lifecycle CO2 reduction of approximately 4000 MT of CO2, while also generating lifecycle cost savings. Other savings may be found in reduced concrete, which generates 1400 kg of CO2 and uses 150 liters of water per cubic yard. The steel rebar has an embodied energy that equates to 4 kg CO2 per kg of structural steel. Other recommendations included reductions in aluminum, which is a lightweight material that was used for fins, curtainwall covers, and other features of the design. A tremendous amount of energy goes into the processing of generating aluminum products from their raw material. As a result, for every kilogram of aluminum produced, we expect emissions of approximately 9.2 kg of CO2. Further research can be given to the total embodied water use in creating construction materials.
While we do not look at CO2 emissions related to anthropogenic efforts, we can look at construction that is labor intensive versus simpler methods as opportunities for water savings. In the project in Africa, local people consume about 4 to 5 Liters of water daily. In their water planning guide, the U.S. Army notes that combat troops in desert climates are consuming approximately 42-58 liters of water per day because of their physical activities. This gives us a glance of what the daily water use of the physical labor workforce in a construction project might look like. When applying this concept of labor into a resource analysis for a desert location, one of the recommendations we developed for our earlier project example explored the use of terracotta rainscreens in lieu of stone. The terracotta is a quarter of the weight of stone and would greatly affect the labor efficiency applied to installation. It would also influence the energy consumed in shipping. Our recommendation resulted in CO2 reductions, but further study on this could also show labor reductions that could also be included with water use reductions.
Not covered, but no less important, are the issues of waste and environmental impacts related to these materials.
Typically, a simple tabular analysis of the key material changes can help demonstrate the value of this measure. The example below shows that a number of contributing systems in a structural analysis drove cost savings and also had profound resource reductions that should be considered as improved strategies from an environmental standpoint.
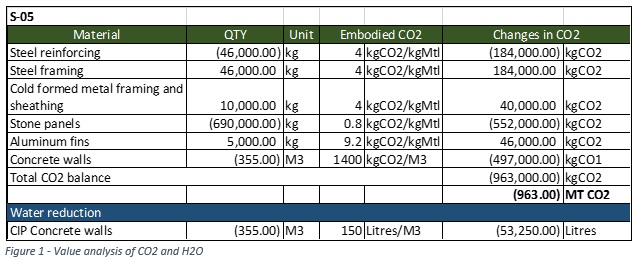
A similar analysis can be applied when calculating energy savings and the lifecycle benefits of a renewable energy measure. In the following example, the team had established the merits of the addition of the photovoltaic energy array but could go further to show the lifecycle carbon impact by applying the regional average electricity CO2 intensity combined with the annual productivity of the array.
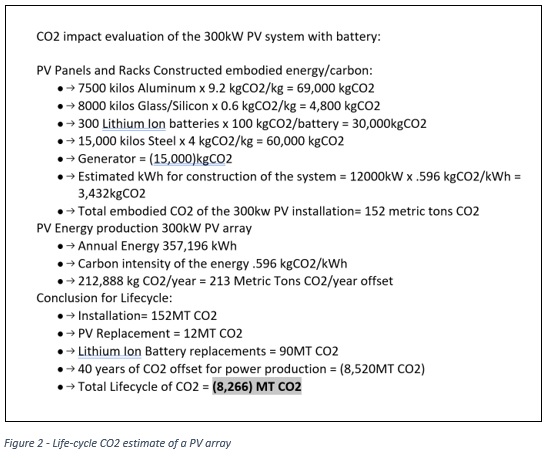
For this example, study and similar studies, VE modelling of these factors were done with the limits of time and scope of the one-week study and were included to demonstrate where a value alternative could create impactful resource reductions that are not seen in cost savings alone and that may have positive political and social impacts.
We have applied these methods on multiple studies, which captured the attention of agency leadership, demonstrating that value engineering could come together with environmental sustainability policy for common benefits. As water, energy, and other resources continue to capture increased economic, social, and political focus and interest, it will become increasingly important to develop data and measurements of these factors for the complete measurement of value.